Network Vulnerability and the Electrical Grid A Deep Dive
Network vulnerability and the electrical grid are intricately linked. Our modern electrical systems rely heavily on interconnected communication networks, making them susceptible to various threats. This exploration delves into the vulnerabilities plaguing these crucial networks, from cyberattacks and physical damage to human error, highlighting the potential consequences of these threats on grid stability and reliability.
The interconnected nature of the electrical grid creates a complex web of potential weaknesses. Understanding these vulnerabilities is critical for developing effective mitigation strategies and ensuring the grid’s resilience against disruptions. From sophisticated cyberattacks to simple human errors, this article examines the multifaceted challenges facing the modern electrical grid and explores innovative solutions to strengthen its defenses.
Introduction to Network Vulnerabilities in the Electrical Grid
The modern electrical grid is a complex network of interconnected power plants, transmission lines, and distribution systems. Its intricate design relies heavily on communication networks to monitor, control, and manage the flow of electricity. This interconnected nature, while crucial for efficiency, also introduces vulnerabilities. Malfunctioning or compromised communication networks can disrupt the entire system, impacting reliability and potentially causing widespread outages.These interconnected systems are vulnerable to various threats, from malicious actors to unforeseen events.
Understanding these vulnerabilities is essential to maintaining grid stability and resilience. The potential consequences of a successful attack can range from localized outages to cascading failures that affect entire regions.
Types of Network Vulnerabilities
The electrical grid’s dependence on communication networks makes it susceptible to a range of threats. These vulnerabilities encompass cyberattacks, physical damage, and human error. Each poses a unique challenge to the grid’s integrity and reliability.
- Cyberattacks: Malicious actors can exploit vulnerabilities in the grid’s communication systems to disrupt operations. These attacks can range from simple denial-of-service attacks to sophisticated intrusions that manipulate control systems. For example, the Stuxnet worm, a sophisticated piece of malware, targeted Iranian nuclear facilities but also highlighted the potential for cyberattacks to affect critical infrastructure like the electrical grid.
- Physical Damage: Physical attacks, such as sabotage or equipment damage, can also disrupt the grid. Storms, floods, or terrorist acts can cause widespread physical damage to power lines, substations, and other critical infrastructure. The 2017 hurricanes in the US, for example, caused significant damage to the power grid, leading to widespread outages.
- Human Error: Human error, whether through negligence, misconfigurations, or inadequate training, can also contribute to vulnerabilities. A simple configuration error in a control system can have devastating consequences, and a lack of security awareness among personnel can lead to unauthorized access.
Consequences of Vulnerabilities
The consequences of these vulnerabilities can be significant, ranging from localized outages to cascading failures that impact entire regions. Compromised control systems can lead to unexpected power fluctuations, equipment damage, and even widespread blackouts. The impact extends beyond immediate disruptions, encompassing economic losses, societal disruption, and potentially even loss of life.
Comparison of Grid Attacks
Attack Type | Description | Impact | Example |
---|---|---|---|
Cyberattack (e.g., Malware) | Exploiting vulnerabilities in software or communication networks to gain unauthorized access and disrupt operations. | System disruptions, data breaches, unauthorized control of equipment, cascading failures. | Stuxnet, targeting Iranian nuclear facilities. |
Physical Damage (e.g., Sabotage) | Direct physical destruction or damage to grid infrastructure. | Localized or widespread outages, equipment failures, complete loss of service. | Acts of vandalism or sabotage targeting power lines or substations. |
Human Error (e.g., Misconfiguration) | Mistakes in system configuration, maintenance, or operation. | Outages, equipment damage, security breaches, unintended consequences. | Improper configuration of a protection relay leading to a false trip. |
Cybersecurity Threats to the Electrical Grid
The modern electrical grid, a complex network of power plants, transmission lines, and distribution systems, is increasingly vulnerable to cyberattacks. These attacks, often motivated by sabotage, espionage, or financial gain, pose a significant threat to the reliability and security of power delivery, potentially causing widespread disruption and economic damage. Understanding the diverse attack vectors and methods used by attackers is crucial for developing effective defense strategies.
Attack Vectors Targeting the Electrical Grid
Cyberattacks targeting the electrical grid can exploit vulnerabilities in various components, from power plants to consumer-facing smart meters. These attacks can disrupt power generation, transmission, or distribution, leading to widespread outages. Attackers often leverage the interconnected nature of the grid, targeting communication networks to gain unauthorized access and control.
Methods of Exploitation in Communication Networks
Attackers employ various methods to exploit vulnerabilities in the grid’s communication networks. These methods often involve phishing campaigns, malware injection, and denial-of-service attacks. Phishing emails or malicious websites can trick employees into revealing sensitive credentials, granting attackers access to critical systems. Malware can infiltrate the network, enabling remote control and data exfiltration. Denial-of-service attacks can overwhelm network resources, preventing legitimate users from accessing vital information or control systems.
Examples of Successful and Failed Attacks, Network vulnerability and the electrical grid
While specific details of successful attacks are often kept confidential for security reasons, documented incidents illustrate the potential consequences. For example, a distributed denial-of-service (DDoS) attack can overload a control center, potentially leading to widespread power outages. The Stuxnet worm, a sophisticated piece of malware, is a notable example of a successful attack targeting industrial control systems, illustrating the potential for large-scale damage.
Conversely, many attempted attacks have failed due to robust security measures and vigilant monitoring.
Impact Assessment of Attack Vectors
Attack Vector | Potential Impact |
---|---|
Phishing | Compromise of credentials, unauthorized access to critical systems, potential for malware injection. |
Malware Injection | Remote control of critical infrastructure, data exfiltration, disruption of operations, potential for physical damage. |
Denial-of-Service Attacks | Interruption of communication, inability to control the grid, potential for cascading failures. |
Advanced Persistent Threats (APTs) | Long-term infiltration, data exfiltration, disruption of operations, potential for sabotage. |
Insider Threats | Malicious actions by authorized personnel, unauthorized access to critical systems, potential for sabotage. |
Physical Vulnerabilities and Threats to the Grid
The electrical grid, a complex network of power plants, transmission lines, and distribution systems, is vulnerable to a range of physical threats. These threats can range from deliberate acts of sabotage to natural disasters, and can have devastating consequences, potentially disrupting power supply to entire regions. Understanding these vulnerabilities is crucial for developing effective security measures.
Physical Infrastructure Vulnerabilities
Physical attacks targeting grid infrastructure can cripple the entire system. These attacks can involve direct damage to equipment, sabotage of control systems, or disruption of critical communication channels. The vulnerability of grid infrastructure to physical threats is amplified by the often remote and isolated locations of many critical components.
Examples of Physical Threats
A multitude of physical threats pose significant risks to the grid’s infrastructure. These include:
- Acts of Sabotage: Deliberate attempts to damage or destroy power lines, substations, or other critical grid components. These acts can be carried out by individuals, groups, or even state-sponsored actors. Examples include targeted bombings or the use of explosives to disrupt transmission lines.
- Natural Disasters: Events such as hurricanes, floods, earthquakes, and wildfires can cause widespread damage to the grid. The destruction of power lines, substations, and transformers can result in extended outages and significant economic losses. For instance, Hurricane Sandy in 2012 caused extensive damage to the US East Coast power grid.
- Terrorist Attacks: Deliberate acts of terrorism targeting critical infrastructure, such as power plants or substations, can have far-reaching consequences, causing widespread disruptions and cascading failures.
- Equipment Malfunctions: Failures of equipment, like transformers or circuit breakers, can lead to localized outages or, if not properly addressed, cascading failures. Corrosion, aging, and inadequate maintenance can all contribute to these failures.
Cascading Failures in the Network
A single physical attack or incident can trigger a cascading failure throughout the grid. The interconnected nature of the grid means that damage to one component can lead to the failure of others. This effect can be amplified by the lack of redundancy in certain areas of the network.
“Cascading failures occur when a disruption in one part of the grid causes a chain reaction, affecting other parts and ultimately leading to widespread outages.”
Physical Threats and their Impact on Grid Components
The following table Artikels potential physical threats and their impact on various grid components:
Physical Threat | Grid Component | Impact |
---|---|---|
Acts of Sabotage | Transmission Lines | Disruption of power flow, potential fire, and cascading failures |
Natural Disasters (Floods) | Substations | Equipment damage, flooding, and short circuits |
Terrorist Attacks | Power Plants | Loss of power generation, possible explosion, and complete grid disruption |
Equipment Malfunctions | Transformers | Overheating, short circuits, and localized outages |
Human Factors and Errors in the Electrical Grid
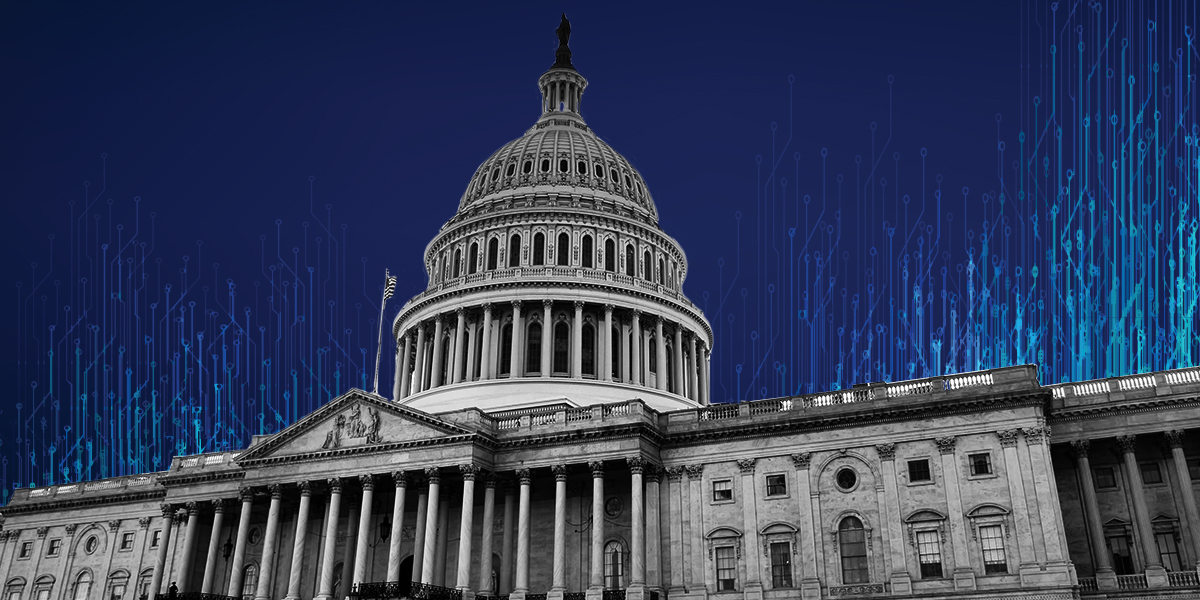
Human error, often underestimated, plays a significant role in creating vulnerabilities in the intricate network of the electrical grid. Operators, maintenance personnel, and even those interacting with the grid remotely can inadvertently introduce risks, compromising security and reliability. These errors can range from simple mistakes in procedures to more complex failures in judgment, highlighting the crucial need for robust training, clear protocols, and proactive oversight to mitigate these risks.The human element represents a critical weak point in security measures designed to protect the electrical grid.
While sophisticated technological safeguards are vital, they are ultimately reliant on the human element for proper implementation and operation. Errors in judgment, miscommunication, fatigue, and insufficient training can bypass even the most advanced security systems, demonstrating the importance of a comprehensive approach to grid security that considers the human factors involved.
Types of Human Errors in the Electrical Grid
Human errors in the electrical grid manifest in various forms. Understanding these different categories is crucial for developing effective mitigation strategies. These errors can range from seemingly minor oversights to major systemic failures, and can have significant impacts on the grid’s overall stability and security.
- Operational Errors: These encompass mistakes in routine tasks, such as incorrect settings, improper procedures, or failures to adhere to established protocols. These errors often stem from inadequate training, insufficient oversight, or a lack of clear communication channels.
- Maintenance Errors: Mistakes during maintenance activities can lead to equipment malfunctions or failures, resulting in unexpected grid disruptions. This can include incorrect repairs, missed inspections, or improper handling of equipment, highlighting the importance of detailed maintenance protocols and rigorous quality control procedures.
- Design Errors: Faulty or incomplete designs can create vulnerabilities in the grid’s architecture. This includes insufficient redundancy, inadequate safety measures, or overlooking potential weaknesses in the system’s design. The grid’s complexity demands careful consideration of these factors during the design phase.
- Judgment Errors: These errors relate to the decision-making process, such as prioritizing immediate tasks over long-term grid stability or misinterpreting critical data. Situations involving limited time, stress, or inadequate information can contribute to poor judgment calls.
- Communication Errors: Ineffective or incomplete communication between personnel can lead to misunderstandings and misinterpretations, causing operational errors or delays in response to critical situations. This underscores the need for clear communication protocols and reliable channels.
Examples of Human Error-Induced Grid Disruptions
Several incidents throughout history have demonstrated the significant impact of human error on the electrical grid. These examples underscore the importance of robust training programs, clear protocols, and a culture of safety within the grid’s operational and maintenance personnel.
- Incorrect settings in automated systems: A change in a single parameter in an automated system can trigger a cascading failure. A misconfigured relay, for instance, can initiate a widespread outage.
- Overlooking critical data: Failure to monitor key metrics or overlooking critical warnings from sensors can lead to the escalation of a small issue into a major outage. This illustrates the need for a vigilant approach to data analysis.
- Inadequate training: Insufficient training on proper procedures and emergency response can lead to delays in handling critical situations. This demonstrates the need for ongoing training and continuous improvement of personnel’s skills.
- Miscommunication between teams: Lack of communication between maintenance and operational teams can lead to unexpected problems and delays in addressing issues.
Categorization of Human Errors and Potential Impact
The table below Artikels various categories of human errors and their potential impact on the electrical grid. This structure facilitates a clear understanding of the diverse ways human error can compromise grid security and reliability.
Category of Error | Description | Potential Impact |
---|---|---|
Operational Errors | Mistakes in routine tasks, improper procedures, or non-adherence to protocols | Local or regional outages, equipment damage, increased risk of cascading failures |
Maintenance Errors | Incorrect repairs, missed inspections, improper handling of equipment | Equipment malfunctions, failures, and potentially large-scale outages |
Design Errors | Insufficient redundancy, inadequate safety measures, or overlooking potential weaknesses in system design | High vulnerability to attacks, reduced resilience to failures, cascading effects |
Judgment Errors | Poor decision-making in critical situations | Delays in response to emergencies, escalation of minor issues, failures to implement preventive measures |
Communication Errors | Ineffective or incomplete communication between personnel | Misinterpretations, delays in resolving issues, missed warnings |
Consequences and Impacts of Grid Disruptions
The electrical grid is the backbone of modern society, powering everything from homes and businesses to hospitals and critical infrastructure. A disruption to this vital network can have catastrophic consequences, impacting not only individuals but also the entire economy and societal well-being. Understanding the potential ramifications of grid outages is crucial for developing effective mitigation strategies.Grid disruptions, whether caused by natural disasters, cyberattacks, or human error, can lead to widespread and long-lasting impacts.
The ripple effect of these disruptions extends far beyond the immediate area affected, creating cascading failures across various sectors. This section will delve into the diverse consequences and impacts of such events.
Economic Losses
Significant economic losses are inevitable following a large-scale grid disruption. Businesses face substantial downtime, leading to lost productivity and revenue. Manufacturing processes halt, supply chains are interrupted, and transportation systems grind to a halt. The costs associated with restoring power and repairing damaged infrastructure are also substantial, potentially burdening national budgets and impacting economic recovery for years.
Public Health Risks
A prolonged grid outage can severely jeopardize public health. Hospitals and medical facilities lose access to life-saving equipment, resulting in delays in treatment and potential loss of life. Access to clean water and sanitation systems is disrupted, creating a breeding ground for disease outbreaks. Food spoilage in refrigerators and freezers can lead to health problems, particularly in vulnerable populations.
The stress and anxiety associated with prolonged power outages can also have adverse effects on mental health.
Societal Impacts
Beyond economic and health concerns, grid disruptions have profound societal impacts. Communication networks are affected, leading to communication breakdowns and difficulties in coordinating emergency responses. Public safety is compromised, and essential services, such as law enforcement and emergency medical services, are hampered. Disruptions to transportation systems create logistical challenges and impede the movement of people and goods.
The disruption of essential services and daily routines can lead to social unrest and create a sense of insecurity.
Cascading Effects
The effects of a grid disruption are not confined to a single sector. The failure of one component can trigger a domino effect, impacting other critical systems. For example, a power outage can disrupt transportation networks, affecting the movement of emergency personnel and supplies. Water treatment plants and sewage systems can also be impacted, creating public health hazards.
The cascading effect can lead to widespread chaos and complicate recovery efforts.
Long-Term Effects
The long-term effects of grid outages extend beyond the immediate aftermath. Damage to infrastructure may require years to repair, and the recovery process can be costly and complex. The economic downturn resulting from the disruption can linger for an extended period, affecting employment rates and overall societal well-being. The experience can also lead to a heightened awareness of the vulnerability of the grid and a need for more robust security measures and resilience strategies.
Examples of Past Grid Disruptions
Numerous historical examples demonstrate the significant consequences of grid disruptions. The Northeast Blackout of 2003, for instance, affected millions of people across the northeastern United States and parts of Canada. This outage highlighted the interconnectedness of the grid and the vulnerability to cascading failures. The California power crisis of 2000-2001 also underscored the importance of balancing supply and demand in a reliable power system.
These and other events have prompted significant improvements in grid management practices, but the threat remains ever-present.
Summary Table: Impacts of Grid Outages
Sector | Impact |
---|---|
Healthcare | Disruption of life-saving equipment, delays in treatment, potential loss of life, water and sanitation issues. |
Economy | Business closures, lost productivity, interrupted supply chains, manufacturing halts, transportation issues. |
Public Safety | Communication breakdowns, difficulty in coordinating emergency responses, impaired law enforcement and emergency medical services. |
Transportation | Disruptions to transportation systems, difficulties in moving people and goods, delays and complications in supply chains. |
Water & Sanitation | Disruption of water treatment and sewage systems, potential health hazards. |
Food Supply | Food spoilage, potential food shortages, impact on food supply chains. |
Communication | Communication breakdowns, difficulty in coordinating emergency responses. |
Mitigation Strategies for Network Vulnerabilities
Protecting the critical infrastructure of the electrical grid from cyberattacks requires a multi-layered approach encompassing various mitigation strategies. This proactive defense involves robust security protocols, physical safeguards, and a culture of vigilance within the grid’s operations. A comprehensive understanding of potential vulnerabilities, coupled with effective mitigation techniques, is crucial for maintaining grid stability and resilience.
Network vulnerabilities in the electrical grid are a serious concern, especially when considering the critical infrastructure involved. Robust cybersecurity measures are essential, but the challenges remain for high-end router suppliers like this one in meeting the demands of such systems. These challenges ultimately impact the overall security of the grid, highlighting the need for innovative solutions to prevent potential attacks.
Security Protocols
Implementing strong security protocols is fundamental to protecting the electrical grid’s network infrastructure. These protocols form the first line of defense against unauthorized access and malicious activity. This includes stringent access controls, robust authentication mechanisms, and regular security audits. Security protocols must be regularly updated to address emerging threats.
- Network Segmentation: Dividing the network into smaller, isolated segments limits the impact of a breach. If one segment is compromised, the others remain protected. This approach is akin to compartmentalizing sensitive data in a corporate network.
- Multi-Factor Authentication (MFA): Requiring multiple forms of verification, such as passwords, security tokens, or biometrics, adds an extra layer of security. This makes it significantly harder for unauthorized individuals to gain access.
- Regular Security Audits: Conducting periodic assessments of the grid’s security posture identifies vulnerabilities and weaknesses before they are exploited. This process should include vulnerability scanning, penetration testing, and security information and event management (SIEM) systems.
- Encryption: Encrypting data transmitted over the grid’s network protects it from eavesdropping and unauthorized modification. This is essential for confidential communications and data exchange.
Physical Protection Measures
Physical security measures are equally important, safeguarding the grid’s physical infrastructure from attacks that can disrupt operations. Physical access control, surveillance, and robust facility security are key elements. These measures work in conjunction with the security protocols to create a comprehensive defense.
Network vulnerabilities in the electrical grid are a serious concern, especially considering the increasing reliance on digital systems. The intricate interplay of software and hardware, much like the complex software battles of the past, like SCO vs IBM, highlights the critical need for robust security measures. For a deeper dive into the historical tech conflicts, check out this fascinating article on sco vs ibm the other reality.
Ultimately, understanding these historical conflicts can help us better anticipate and mitigate future vulnerabilities in the modern electrical grid.
- Physical Access Control: Implementing strict access controls to physical facilities housing grid equipment prevents unauthorized personnel from gaining access to sensitive areas. This can include security personnel, surveillance cameras, and controlled entry points.
- Surveillance Systems: Employing advanced surveillance technologies, including cameras and sensors, monitors physical access points and detects suspicious activity. This helps in identifying and responding to potential threats promptly.
- Robust Facility Security: Protecting grid facilities from physical attacks, such as sabotage or vandalism, is critical. This includes reinforced structures, security fencing, and alarm systems.
Other Defensive Strategies
Beyond security protocols and physical protection, other defensive strategies are crucial for grid resilience. These strategies include incident response planning, employee training, and collaboration with law enforcement and industry partners. A comprehensive approach that integrates these elements is essential for effective mitigation.
- Incident Response Planning: Developing and testing incident response plans ensures that the grid has a structured approach to handle security breaches and disruptions. This includes clear communication channels, escalation procedures, and recovery strategies.
- Employee Training: Educating personnel on cybersecurity threats and best practices is vital. Training should cover recognizing suspicious activities, reporting potential vulnerabilities, and adhering to security protocols.
- Collaboration: Working with law enforcement agencies and industry partners allows for information sharing, threat intelligence, and joint responses to potential attacks. Collaborative efforts strengthen the overall security posture.
Mitigation Strategies Effectiveness Table
Mitigation Strategy | Description | Effectiveness (High/Medium/Low) | Examples |
---|---|---|---|
Network Segmentation | Dividing network into smaller segments | High | Reduces impact of a breach |
Multi-Factor Authentication | Requiring multiple authentication factors | High | Increases security against unauthorized access |
Physical Access Control | Restricting physical access to facilities | Medium | Prevents unauthorized personnel access |
Surveillance Systems | Monitoring physical access points | Medium | Detects suspicious activities |
Proactive Security Measures
Proactive security measures are crucial for maintaining a resilient electrical grid. These measures anticipate potential threats and implement preventative strategies. These include continuous monitoring, threat intelligence, and vulnerability management.
- Continuous Monitoring: Constantly monitoring the grid’s network and systems for anomalies helps in identifying potential threats early. Advanced tools and techniques can detect unusual patterns and behavior.
- Threat Intelligence: Staying informed about emerging threats and vulnerabilities through industry reports, security advisories, and threat intelligence feeds is critical. This allows for proactive measures to mitigate potential threats.
- Vulnerability Management: Regularly identifying, assessing, and mitigating vulnerabilities in the grid’s network and systems is essential. This includes patch management, software updates, and security hardening.
Advanced Technologies and Solutions for Enhancing Grid Security
The electrical grid, a critical infrastructure, faces evolving threats demanding innovative security measures. Advanced technologies offer a powerful arsenal to bolster grid resilience and enhance the detection and response capabilities against cyber and physical attacks. These technologies, ranging from artificial intelligence to blockchain, can be integrated into existing systems to fortify the grid’s overall security posture.Modernizing the grid’s security architecture is not just about reacting to threats; it’s about proactively anticipating and mitigating risks.
This proactive approach necessitates a multi-faceted strategy that integrates advanced technologies with existing infrastructure to create a more resilient and secure system.
Role of Artificial Intelligence (AI) in Grid Security
AI algorithms can analyze vast amounts of data from various grid components, such as sensors and meters, to identify anomalies and potential threats in real-time. This predictive capability is crucial for preventing outages and minimizing disruptions. For instance, AI-powered systems can detect subtle patterns indicative of equipment malfunctions before they escalate into major failures.
Network vulnerabilities in the electrical grid are a serious concern, impacting everything from power outages to potential cyberattacks. Imagine the disruption if a sophisticated attack targeted the grid, potentially crippling essential services. Thankfully, innovative technologies like the new Sharp Mebius PC RD3D laptop with 3D display here might help us visualize and analyze these vulnerabilities more effectively, ultimately bolstering grid security.
This advancement in 3D displays could be a game-changer for understanding and mitigating network vulnerabilities in the electrical grid, paving the way for a more resilient system.
Machine Learning for Enhanced Threat Detection
Machine learning (ML) algorithms can be trained to recognize and categorize various cyber threats. By analyzing historical data and identifying patterns, ML models can significantly improve the accuracy and speed of threat detection. This approach is particularly effective in identifying zero-day attacks that bypass traditional security measures.
Blockchain Technology for Secure Data Management
Blockchain’s decentralized and immutable nature can be leveraged to enhance the security and transparency of grid data. Secure transactions and data integrity are essential in a system where data from numerous sources must be accurately transmitted and verified. Implementing blockchain can enhance trust and confidence in the data exchange process.
Implementation Strategies for Advanced Technologies
Implementing these advanced technologies requires careful planning and integration. The process should include data standardization across different grid components, ensuring compatibility with existing infrastructure, and prioritizing areas with the highest risk. Furthermore, cybersecurity protocols need to be designed to protect the data used by these advanced systems.
Table of Advanced Technologies and Their Applications in Grid Security
Technology | Application in Grid Security |
---|---|
Artificial Intelligence (AI) | Predictive maintenance, anomaly detection, real-time threat assessment, optimized resource allocation |
Machine Learning (ML) | Automated threat classification, advanced pattern recognition, improved response time to cyber threats, enhanced fault detection |
Blockchain | Secure data management, tamper-proof transaction records, improved transparency and trust in data exchange, enhanced grid authentication |
Case Studies of Network Vulnerabilities in the Electrical Grid: Network Vulnerability And The Electrical Grid
Unveiling the vulnerabilities of the electrical grid’s intricate network is crucial for strengthening its resilience. Real-world case studies provide valuable insights into past incidents, enabling us to understand the root causes, consequences, and ultimately, develop effective mitigation strategies. These studies highlight the critical need for continuous improvement and proactive security measures to prevent future disruptions.
Specific Examples of Grid Cyberattacks
Analyzing past incidents reveals a range of vulnerabilities exploited by cyber attackers. These incidents underscore the interconnectedness of modern grids and the potential for cascading failures. Understanding the methods used in these attacks allows for the development of stronger defenses.
Case Study | Root Cause | Consequences | Lessons Learned | Investigation Methodology |
---|---|---|---|---|
The 2015 Ukraine Power Grid Attack | A sophisticated malware attack exploited known vulnerabilities in the SCADA system, disrupting operations for hours. | Significant power outages, economic losses, and disruption of critical services. The attack exposed the vulnerability of the grid’s critical infrastructure to cyberattacks. | The attack highlighted the importance of robust cybersecurity protocols and the need for real-time monitoring and incident response capabilities. The incident emphasized the need for enhanced network segmentation to limit the impact of potential attacks. | Forensic analysis of compromised systems, network traffic logs, and interviews with affected personnel. |
The 2016 US Power Grid Cyber Incident | Unknown attackers targeted a power grid company, attempting to gain unauthorized access. The attackers leveraged a phishing campaign to compromise employee accounts. | While the incident did not result in a complete outage, the attack demonstrated the potential for significant disruption through targeted social engineering. | The incident emphasized the importance of employee training on cybersecurity awareness and the need for strong authentication measures. The incident highlighted the effectiveness of phishing campaigns and the need for proactive defense strategies against social engineering tactics. | Analysis of network logs, security alerts, and incident reports, including information gathered from the affected personnel. |
A Hypothetical Grid Attack Focused on Smart Meters | A malicious actor gains access to a smart meter network, and uses it to manipulate power flow or disrupt communication protocols. | Potential consequences could include unexpected power surges, reduced grid stability, and difficulties in load balancing. | This hypothetical scenario emphasizes the vulnerability of smart grid technologies to unauthorized access. It demonstrates the need for robust security measures for smart meters, including encryption and secure communication protocols. | Simulation modeling of the attack, penetration testing of smart meter systems, and analysis of potential impact on the broader grid. |
Investigative Methodologies for Cyberattacks
Thorough investigations are essential for understanding the nature and extent of grid cyberattacks. This includes examining network logs, system configurations, and security alerts. The specific methodologies applied depend on the type of attack and the affected infrastructure.
- Network Forensics: Analyzing network traffic logs, security event logs, and system configurations to identify anomalies and the pathways used by attackers.
- Vulnerability Assessment: Identifying potential weaknesses in the grid’s network infrastructure and systems. This involves scanning systems for known vulnerabilities and evaluating the impact of exploitation.
- Incident Response Planning: Having pre-defined procedures to deal with cyber incidents, including containment, eradication, recovery, and post-incident analysis. This involves training and drills to prepare for various scenarios.
- Threat Intelligence Gathering: Monitoring for emerging threats and attack vectors. This includes analyzing threat actor motivations, capabilities, and tactics. Using threat intelligence from various sources and analyzing trends to improve defensive strategies is critical.
Final Summary
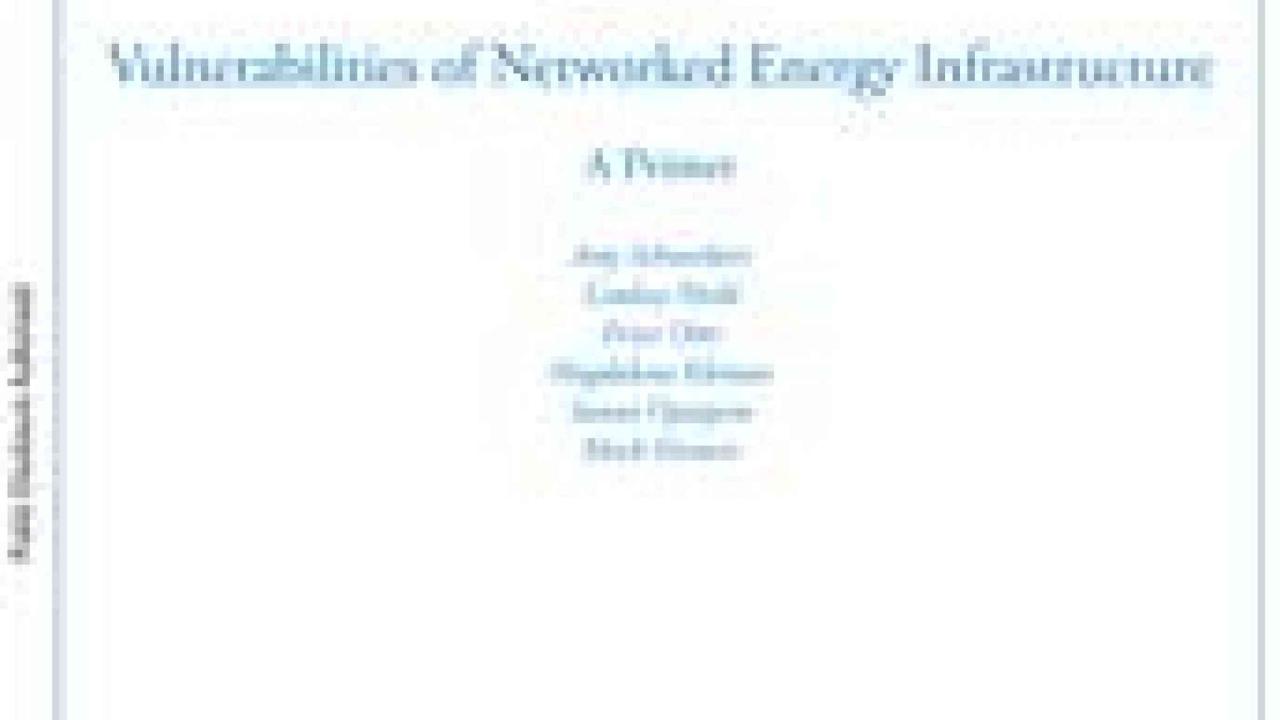
In conclusion, network vulnerability and the electrical grid present a multifaceted challenge demanding a multifaceted solution. This discussion has explored the various threats, from cyberattacks to physical damage and human error, highlighting the critical need for robust security measures. Ultimately, the future of a stable and reliable electrical grid hinges on our collective ability to understand these vulnerabilities and implement proactive mitigation strategies, incorporating advanced technologies and a deep understanding of human factors.
We must continue to adapt and innovate to ensure the continued operation of this vital infrastructure.