Earth Distorts Time Einsteins Prediction
As einstein predicted earth distorts time space – As Einstein predicted, Earth distorts time and space. This fascinating concept, a cornerstone of general relativity, describes how massive objects warp the fabric of spacetime. We’ll explore the theory behind this phenomenon, delving into the key postulates of special and general relativity, and examining the experimental evidence that confirms Einstein’s groundbreaking prediction. From the basics of spacetime curvature to the practical implications for GPS technology, this exploration will unravel the mysteries of how mass affects time itself.
Einstein’s theory revolutionized our understanding of gravity, proposing that massive objects create a curvature in spacetime, rather than exerting a force. This curvature dictates the motion of objects, including light. This is in contrast to Newton’s view of gravity as a force acting at a distance. We will delve into the detailed differences between these two models and the supporting experimental evidence.
Introduction to the Theory of Relativity: As Einstein Predicted Earth Distorts Time Space
Einstein’s theory of relativity revolutionized our understanding of gravity, space, and time. It fundamentally altered the way we perceive the universe, moving beyond the classical Newtonian framework. This theory isn’t just a set of equations; it’s a new way of thinking about the very fabric of reality. It’s a framework that encompasses both the large-scale structure of the cosmos and the behavior of objects at the atomic level, offering profound insights into the universe’s workings.The theory is divided into two parts: special relativity and general relativity.
Special relativity deals with the relationship between space and time for observers in uniform motion, while general relativity expands this framework to include accelerating observers and gravity. Both theories share a core principle: the laws of physics are the same for all observers in uniform motion. This seemingly simple statement has profound implications, leading to surprising predictions about the behavior of light, time, and gravity.
Key Postulates of Special Relativity
Special relativity is based on two postulates:
- The laws of physics are the same for all observers in uniform motion.
- The speed of light in a vacuum is constant for all observers, regardless of the motion of the light source.
These postulates, seemingly simple, lead to remarkable consequences, including time dilation and length contraction.
Einstein’s theory about Earth warping spacetime is fascinating, but even more mind-blowing is how superpowered PDAs are challenging the dominance of laptops. These handheld marvels, packed with processing power previously unimaginable, are pushing the boundaries of computing. They’re not just smaller, faster versions of laptops; they’re a completely new paradigm. This evolution mirrors the way Einstein’s theories revolutionized our understanding of the universe, showing how seemingly disparate ideas can impact our world.
The implications for our future in terms of portability and computing power are truly profound, just like Einstein’s prediction of time distortion. Check out this article to learn more about superpowered pdas challenge the laptop platform for a deeper dive into this technology revolution. Ultimately, both advancements demonstrate how our understanding of reality, and its potential, can be rewritten.
Key Postulates of General Relativity
General relativity extends special relativity to include accelerating observers and gravity. It posits that gravity isn’t a force, but rather a curvature of spacetime caused by mass and energy.
- The equivalence principle: Gravity is indistinguishable from acceleration. An observer in a closed elevator accelerating upwards experiences the same effects as an observer in a gravitational field.
- The geodesic principle: Objects move along the “straightest” possible paths in curved spacetime. These paths, called geodesics, are not necessarily straight lines in the everyday sense, but rather curves determined by the curvature of spacetime.
Newtonian vs. Einsteinian Gravity
Newton’s law of universal gravitation describes gravity as a force of attraction between objects with mass. Einstein’s theory of general relativity, on the other hand, describes gravity as a curvature of spacetime.
Characteristic | Newtonian Gravity | General Relativity |
---|---|---|
Nature of Gravity | A force acting instantaneously across distances. | A curvature of spacetime caused by mass and energy. |
Speed of Gravity | Instantaneous. | Finite, the speed of light. |
Description of Orbits | Circular or elliptical orbits around a central mass. | Geodesics in curved spacetime, potentially exhibiting precession or other complex trajectories. |
Description of Light | Not affected by gravity. | Affected by gravity due to the curvature of spacetime. |
Explanation of Mercury’s Perihelion Precession | Fails to explain the observed precession of Mercury’s orbit. | Accurately predicts the precession. |
Newtonian gravity provides an excellent approximation for many everyday situations, but it fails to account for certain phenomena, particularly at high speeds and strong gravitational fields. General relativity, while more complex, provides a more accurate and complete description of gravity in these situations.
Examples of General Relativity in Action
General relativity’s predictions have been experimentally verified in numerous ways. The bending of starlight around massive objects, like the Sun, is a direct consequence of spacetime curvature. Gravitational time dilation, where time passes slower in stronger gravitational fields, has been observed in experiments involving atomic clocks at different altitudes. These observations confirm the validity of Einstein’s theory.
GPS systems, for example, rely on extremely precise timekeeping. The accuracy of these systems depends critically on accounting for both special and general relativistic effects.
The Distortion of Spacetime by Mass
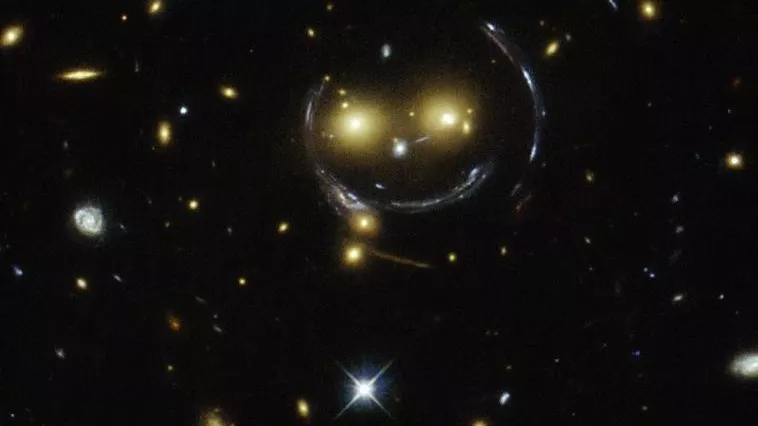
Einstein’s General Theory of Relativity revolutionized our understanding of gravity, not as a force, but as a curvature of spacetime. Imagine spacetime as a fabric, a sheet stretched taut. Placing a heavy object on this sheet causes it to dip or curve. This distortion is what we perceive as gravity. Objects moving near the massive object are deflected from their straight-line paths, appearing to be pulled towards it.The curvature of spacetime isn’t just a theoretical concept; it’s a measurable phenomenon.
Observations of light bending around massive objects, like stars, provide crucial evidence for this theory. Furthermore, the theory explains the precession of Mercury’s orbit, a phenomenon that Newtonian gravity couldn’t account for. This warping of spacetime has profound implications for our understanding of the universe.
Spacetime Curvature and Visualization
Spacetime curvature is a fundamental concept in General Relativity. It describes how the presence of mass and energy warps the fabric of spacetime, causing objects to follow curved paths. Visualizing this can be challenging, but one common analogy is a bowling ball placed on a stretched rubber sheet. The bowling ball creates a dip, and if you roll a marble nearby, it will curve towards the bowling ball.
Similarly, massive objects like planets and stars create a curvature in spacetime, influencing the trajectories of other objects. This curvature is not limited to two dimensions; it exists in four dimensions, including time.
Effects of Massive Objects on Spacetime Geometry
Massive objects, regardless of their composition, warp the surrounding spacetime. The degree of curvature depends directly on the object’s mass. The more massive the object, the greater the curvature. This warping affects not only the paths of objects moving through spacetime but also the propagation of light. This effect is directly proportional to the mass and inversely proportional to the distance from the object.
Light, as it travels through spacetime, follows the curves created by massive objects.
Examples of Mass Distorting Spacetime
- Earth: Earth’s mass creates a noticeable but relatively gentle curvature of spacetime around it. This curvature is responsible for the gravitational pull we experience on Earth’s surface. The curvature is so small that we don’t notice it in everyday life, but it’s crucial for understanding orbital mechanics.
- A Star: A star’s significantly greater mass than Earth results in a much more pronounced curvature of spacetime. The stronger gravitational field affects the trajectories of planets orbiting the star and the paths of light passing nearby. This curvature is noticeable in astronomical observations, such as gravitational lensing, where light from distant objects is bent by the intervening star’s gravitational field.
- A Black Hole: A black hole, with its immense mass concentrated in an incredibly small region, creates an extreme curvature of spacetime. The curvature is so strong that nothing, not even light, can escape from within the event horizon. This extreme distortion is caused by the black hole’s singularity, a point of infinite density. The spacetime around a black hole is warped to an extreme degree, creating a gravitational well from which nothing can return.
Illustrative Table of Spacetime Distortion Scenarios
Object | Mass | Spacetime Distortion | Observable Effects |
---|---|---|---|
Earth | Moderate | Relatively small curvature | Orbital motion of satellites, gravitational pull on objects |
Star | High | Significant curvature | Gravitational lensing, orbital dynamics of planets |
Black Hole | Extreme | Extreme curvature; singularity | Light bending, no escape from event horizon |
Evidence for Gravitational Time Dilation
Einstein’s theory of general relativity proposes that gravity affects the passage of time. This concept, known as gravitational time dilation, predicts that time passes slower in stronger gravitational fields. This seemingly counterintuitive idea has been experimentally verified, providing compelling evidence for the theory’s accuracy. The experiments demonstrating this effect are crucial in understanding the universe at both large and small scales.
Experimental Verification of Gravitational Time Dilation
Numerous experiments have confirmed the prediction of gravitational time dilation. These experiments typically involve comparing the rate of timekeeping devices at different altitudes or in varying gravitational potentials. The subtle differences in the passage of time, though small, are measurable with precise instruments.
The Hafele-Keating Experiment
The Hafele-Keating experiment, conducted in 1971, is a classic example of testing gravitational time dilation. This experiment involved flying atomic clocks around the Earth on commercial jets. The clocks were carefully synchronized before the flights.
- The experiment demonstrated that time passes slightly faster for the clocks on the moving planes compared to the stationary clocks on Earth.
- This difference in time is attributable to both special relativistic and general relativistic effects.
- The results aligned remarkably well with the predictions of general relativity, confirming the theory’s validity in practical scenarios.
The Pound-Rebka Experiment
The Pound-Rebka experiment, performed in the 1950s, offered an alternative approach to verify gravitational time dilation. This experiment used the principle of nuclear gamma-ray resonance.
- Gamma rays were emitted from a source at a higher altitude and detected at a lower altitude.
- The experiment measured a slight decrease in the gamma ray’s frequency, directly related to the gravitational potential difference.
- The frequency shift corresponded precisely to the predicted gravitational time dilation, providing further support for general relativity.
Comparison and Contrasting Results
The Hafele-Keating and Pound-Rebka experiments, while using different methodologies, both yielded results that matched general relativity’s predictions for gravitational time dilation. The agreement demonstrates the theory’s predictive power and its ability to describe the intricate relationship between gravity and time. Both experiments were carefully designed to minimize extraneous factors, ensuring that the observed effects were indeed due to gravitational time dilation.
Experimental Findings Table
Experiment | Methodology | Results | Comparison with GR Predictions |
---|---|---|---|
Hafele-Keating | Atomic clocks flown on jets at different altitudes and speeds | Moving clocks ran slightly faster than stationary clocks. | Precisely aligned with GR predictions, demonstrating both special and general relativistic effects. |
Pound-Rebka | Measuring the frequency shift of gamma rays emitted between different altitudes. | Observed a decrease in frequency of gamma rays, consistent with a time dilation effect. | Results matched GR predictions, providing a different approach to verify the effect. |
Implications of Earth’s Distortion of Spacetime
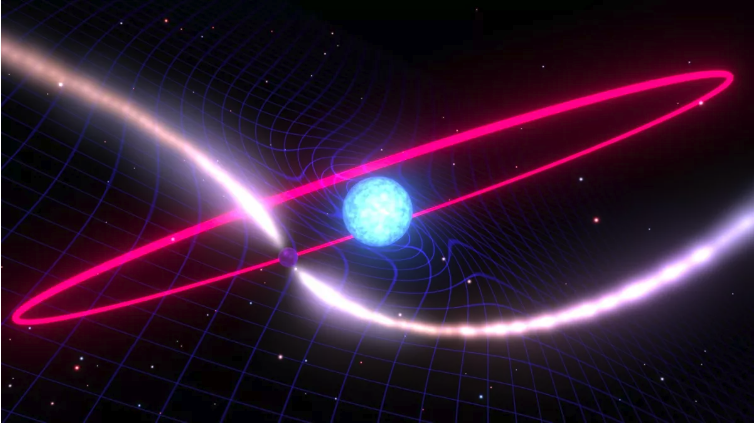
Einstein’s theory of general relativity paints a fascinating picture of gravity not as a force, but as a curvature of spacetime. This curvature, influenced by mass and energy, has profound implications for everyday phenomena, even on a seemingly mundane scale like the Earth’s surface. Understanding how Earth warps spacetime is crucial for comprehending technologies like GPS, highlighting the intricate dance between theory and practical application.Earth’s mass warps the fabric of spacetime, creating a gravitational field.
This warping isn’t just theoretical; it has tangible effects, most notably impacting the passage of time itself. This effect, known as gravitational time dilation, is subtle but measurable, and its consideration is essential for accurate timekeeping, particularly in global positioning systems.
GPS Systems and the Necessity of General Relativity
GPS satellites orbit high above Earth, experiencing a weaker gravitational field than those on the surface. This difference in gravitational strength leads to a difference in the rate at which time passes for the satellites compared to clocks on the ground. Furthermore, the satellites are moving at high speeds, which also affects their internal clocks. Both effects, special and general relativistic, need to be accounted for to ensure accurate positioning.
Gravitational Time Dilation in GPS
The difference in the rate of time between the clocks on the satellites and those on Earth is not negligible. These discrepancies, stemming from both special and general relativity, must be precisely accounted for in GPS systems to maintain their accuracy. Without these corrections, GPS systems would accumulate errors over time, leading to significant inaccuracies in positioning.
Corrections for GPS Signals, As einstein predicted earth distorts time space
Source of Error | Correction | Magnitude of Effect (per day) |
---|---|---|
Special Relativity (Satellite Velocity) | Faster time on the satellite. | 7 microseconds |
General Relativity (Weaker Gravitational Field) | Slower time on the satellite. | 45 microseconds |
Total Correction | Combined effect of special and general relativity. | 38 microseconds |
The table above summarizes the corrections required for GPS signals due to gravitational time dilation. These corrections, seemingly minute, are vital for the precision of GPS. If these corrections were omitted, GPS positions would drift by several kilometers each day. This highlights the remarkable precision required in modern technologies and the fundamental importance of Einstein’s theories.
Einstein’s theory about Earth warping spacetime is fascinating, right? Well, it turns out that the intricacies of how that happens are constantly being refined. Check out this recent update on Longhorn exposed at the Windows Developer Conference update longhorn exposed at windows developer conference for a glimpse into the cutting-edge tech behind how we’re exploring the relationship between technology and these fundamental physics principles.
It’s amazing how the search for deeper understanding in areas like this often touches on completely different fields like software development! Ultimately, understanding the universe, including how time and space interact, remains a profound challenge.
Advanced Concepts and Applications
Einstein’s theory of general relativity, far from being a mere academic exercise, has profound implications for our understanding of the cosmos. It unveils the dynamic nature of spacetime, revealing how massive objects warp this fabric and influencing the trajectories of light and matter. This warping gives rise to some of the most fascinating and extreme phenomena in the universe, such as black holes and gravitational waves.This exploration delves into these advanced concepts, illustrating their significance and how they are applied in various astrophysical contexts.
We will examine the formation of black holes, their characteristics, and the profound impact of gravitational waves on our comprehension of the universe’s evolution.
Einstein’s theory, famously predicting that massive objects warp spacetime, is a fascinating concept. Think of Earth as a bowling ball on a stretched sheet – it creates a dip, much like how gravity affects the fabric of spacetime. This, however, is fundamentally different from the complexities of internet infrastructure, as highlighted in Verisign’s claim that their SiteFinder service, for instance, does not destabilize the internet’s foundation.
Verisign claims SiteFinder does not undermine stability of the net. Ultimately, while the internet’s intricacies might seem complex, the fundamental principles of gravity and spacetime distortion, as predicted by Einstein, still hold true.
Black Holes: Gravitational Prisons
Black holes are regions of spacetime where gravity is so strong that nothing, not even light, can escape. They are formed from the remnants of massive stars that have collapsed under their own gravity after exhausting their nuclear fuel. This collapse compresses the star’s mass into an incredibly small volume, generating a singularity at the center where the known laws of physics break down.
Formation of Black Holes
The life cycle of a massive star is crucial to understanding black hole formation. When a star much more massive than our Sun exhausts its nuclear fuel, it can no longer support itself against its own gravity. The core collapses catastrophically, causing an implosion that results in a black hole. The critical mass required for this collapse is significant, often many times the mass of our Sun.
Supernova explosions, the dramatic deaths of massive stars, are often associated with the formation of black holes. The remnant core, if sufficiently massive, will continue to collapse under its own gravity.
Gravitational Waves: Ripples in Spacetime
Gravitational waves are ripples in the fabric of spacetime that propagate outward from sources of strong gravitational acceleration. They are predicted by Einstein’s theory of general relativity and are generated by violent cosmic events, such as colliding black holes or merging neutron stars. These waves travel at the speed of light, carrying information about the cataclysmic events that created them.
Astrophysical Applications
The study of gravitational waves is revolutionizing astrophysics. By detecting these waves, scientists can gain insights into the universe’s most extreme events, including the merging of black holes and neutron stars. Observations of these events provide crucial data about the properties of these objects and the fundamental forces at play in the universe. Furthermore, they provide a unique tool for testing Einstein’s theory of general relativity in extreme environments.
These observations can also provide insights into the distribution of dark matter and dark energy.
Types of Black Holes and Their Characteristics
Type of Black Hole | Formation | Characteristics |
---|---|---|
Stellar Black Holes | Collapse of massive stars | Relatively smaller, typically a few to tens of solar masses. |
Supermassive Black Holes | Growth from accretion of matter | Found at the centers of galaxies, millions to billions of solar masses. |
Intermediate-Mass Black Holes | Formation mechanism not yet fully understood | Occupy a mass range between stellar and supermassive black holes, from hundreds to thousands of solar masses. |
Visual Representation of Spacetime Distortion
Einstein’s theory of general relativity revolutionized our understanding of gravity, describing it not as a force but as a curvature of spacetime. Visualizing this curvature, though impossible in its full four-dimensional form, is crucial for grasping the implications of this revolutionary theory. These visualizations help us imagine how mass warps the fabric of the universe, affecting the paths of light and objects.Understanding spacetime distortion requires moving beyond our intuitive three-dimensional experience.
We must imagine a four-dimensional continuum where time is the fourth dimension, intertwined with the three spatial dimensions. Mass, in this framework, acts as a sculptor, molding this spacetime into a warped and curved form.
Visualizing Curvature around Massive Objects
Visualizations of spacetime distortion typically depict massive objects, like Earth, as creating a dip or well in a two-dimensional representation of spacetime. This “well” is a visual representation of how the object warps the fabric around it. Imagine a bowling ball placed on a stretched rubber sheet; the ball creates a dip, and any small object rolling nearby will curve towards the ball due to the dip.
Similarly, planets and stars create a curvature in spacetime, affecting the paths of objects and light within their gravitational influence.
Representing the Effect on Light Paths
Light, despite being massless, follows the curves of spacetime. Visualizations often show light rays bending as they pass near a massive object. This bending is a direct consequence of the curvature of spacetime, a prediction of general relativity that has been experimentally verified. Imagine light as a marble rolling across the same rubber sheet with the bowling ball; the marble’s path will curve towards the ball due to the dip.
Similarly, starlight passing near the Sun is deflected by the Sun’s gravitational field.
Methods for Visualization
Visualizations can take several forms. Two-dimensional representations, such as a rubber sheet analogy, provide a simplified understanding of the concept, but fail to capture the full four-dimensional nature of spacetime. Three-dimensional models, though more complex, offer a more accurate representation of the warped spacetime around an object. Computer-generated simulations can depict the distortion of spacetime around massive objects, highlighting the effects on light and other objects.
Examples of Visualizations
“A visualization of spacetime curvature around Earth might show Earth as a sphere, and the surrounding spacetime as a warped sheet, with the warp increasing in intensity closer to the Earth.”
These visualizations can also incorporate time as a fourth dimension, although this is challenging to represent visually. Visualizations of the bending of starlight passing near the Sun demonstrate the distortion of spacetime by the Sun’s immense mass. The extent of the distortion depends on the mass of the object; more massive objects create a greater curvature. Furthermore, these visualizations are not just theoretical; they are based on Einstein’s equations and have been confirmed by astronomical observations.
Last Word
In conclusion, Einstein’s prediction of Earth’s influence on spacetime is remarkably profound. The distortion of spacetime isn’t just a theoretical concept; it has tangible consequences, affecting everything from the accuracy of GPS systems to our understanding of the universe’s most extreme phenomena like black holes. The exploration of this fundamental principle continues to shape our comprehension of the cosmos and our place within it.