Life After Moores Law Beyond Silicon
Life after moores law beyond silicon – Life after Moore’s Law: Beyond Silicon explores the exciting and potentially revolutionary shift away from silicon-based computing. This journey delves into alternative materials, redefining computing paradigms, and envisioning the future of this transformative technology. From graphene and carbon nanotubes to quantum dots, we’ll examine the potential benefits and drawbacks of these emerging technologies, while exploring the challenges and opportunities ahead.
The rapid pace of technological advancement is driving innovation in every sector. The limitations of silicon chips are prompting a search for new, more efficient materials and computing architectures. This exploration will cover a wide range of topics, including the comparison of various alternative materials, the implications of new computing paradigms, and potential applications across various industries. The future of computing is ripe with possibilities, and this deep dive will provide insights into the transformative changes that are on the horizon.
Beyond Silicon
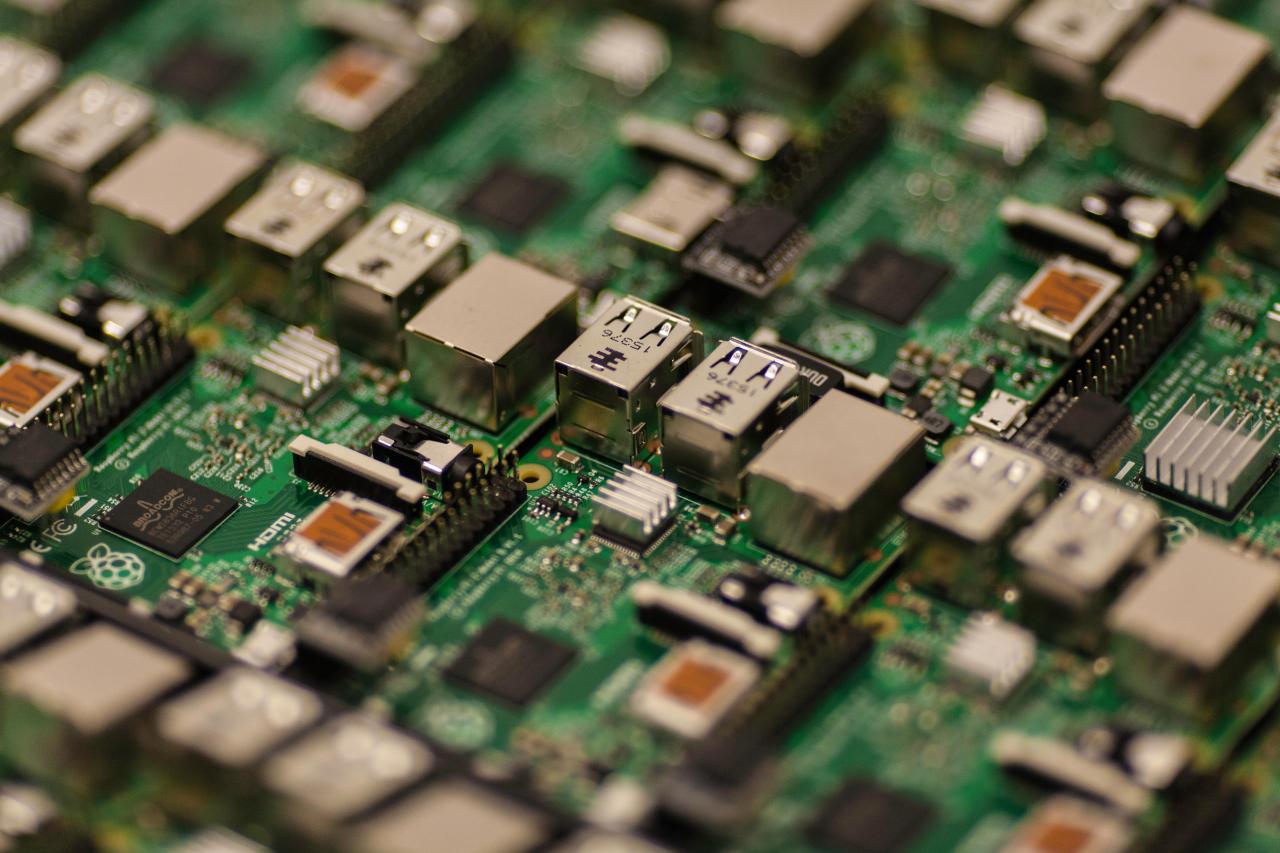
The relentless march of Moore’s Law, while instrumental in driving technological advancement, is facing a fundamental constraint: the limitations of silicon-based transistors. As transistors shrink, quantum effects and material imperfections become increasingly significant, hindering further performance gains. This necessitates a shift towards alternative materials and architectures for computing, promising to push the boundaries of processing power and energy efficiency.Alternative materials offer a tantalizing array of possibilities to overcome these limitations.
They exhibit unique electrical and mechanical properties that could potentially lead to revolutionary improvements in computing performance and energy consumption. This exploration of alternatives, therefore, is crucial for the future of computing.
Alternative Materials and Technologies
A wide range of materials beyond silicon are being investigated for their potential in computing applications. These include materials with exceptional conductivity, high mobility, and unique quantum properties. The search for alternatives is driven by the need for improved performance and energy efficiency, especially as existing silicon-based technologies reach their physical limits.
Graphene
Graphene, a single layer of carbon atoms arranged in a honeycomb lattice, exhibits exceptional electrical conductivity and mechanical strength. Its potential advantages include high electron mobility, enabling faster switching speeds in transistors, and potentially lower power consumption compared to silicon. However, challenges exist in achieving reliable device fabrication and overcoming the limitations in achieving stable and scalable manufacturing processes.
Graphene’s inherent flexibility also presents potential manufacturing difficulties.
Carbon Nanotubes
Carbon nanotubes, cylindrical structures composed of carbon atoms, possess extraordinary mechanical and electrical properties. Their potential benefits include high electron mobility, thermal conductivity, and potentially improved transistor performance. However, challenges remain in controlling the alignment and uniformity of nanotubes during fabrication. The scalability of nanotube-based devices is also a significant hurdle, along with the development of reliable manufacturing processes.
Quantum Dots, Life after moores law beyond silicon
Quantum dots, semiconductor nanocrystals, exhibit unique quantum mechanical properties that could enable novel computing architectures. Their potential advantages include tunable optical and electrical properties, potentially leading to high-density and energy-efficient devices. Challenges include controlling the size and shape of quantum dots during synthesis, ensuring consistent properties across large-scale production, and the development of suitable integration methods.
Other Materials
Other promising materials under investigation include various 2D materials (beyond graphene), such as transition metal dichalcogenides (TMDs), which show promise in different computing applications, with unique optical and electronic properties. Each material presents unique advantages and disadvantages, demanding tailored fabrication processes and design considerations.
Comparative Analysis
Material | Properties | Potential Applications | Advantages | Disadvantages |
---|---|---|---|---|
Silicon | Abundant, mature fabrication processes | Current integrated circuits | Established technology, cost-effective | Reaching performance limits, high power consumption |
Graphene | High electron mobility, high strength | High-speed transistors, flexible electronics | Potential for faster and more energy-efficient devices | Scalability and manufacturing challenges |
Carbon Nanotubes | High electron mobility, high thermal conductivity | High-performance transistors, thermal management | Potential for improved performance and thermal control | Control over alignment, scalability issues |
Quantum Dots | Tunable optical and electrical properties | Quantum computing, novel sensing devices | Potential for highly integrated devices, tunable properties | Controlling size and shape, manufacturing complexity |
Current Research and Development
Significant research efforts are underway to explore the practical applications of these alternative materials. Numerous research groups and companies are actively investigating various aspects of material synthesis, device fabrication, and circuit design. Examples include academic collaborations and industry initiatives focusing on specific material classes and applications.
Redefining Computing Paradigms
The relentless march of technological advancement beyond Moore’s Law necessitates a profound reimagining of computing paradigms. Current silicon-based architectures, while remarkably successful, are reaching their physical limits. This compels us to explore alternative approaches, pushing the boundaries of computation and potentially unlocking unprecedented capabilities. New materials, novel architectures, and innovative programming models are crucial to this evolution. The transition will not be seamless, but rather a fundamental shift in how we think about computation itself.The limitations of silicon-based transistors, such as power consumption and scaling limitations, are driving the exploration of alternative materials and architectures.
This quest for improved performance, reduced energy consumption, and enhanced computational capabilities is fueling the development of entirely new computing paradigms. The potential rewards are immense, promising advancements in artificial intelligence, scientific simulations, and countless other applications.
Potential Shifts in Computing Architecture
Traditional Von Neumann architecture, with its separation of memory and processing units, is proving increasingly inefficient for complex tasks. Emerging architectures, such as neuromorphic computing and quantum computing, aim to overcome these limitations by mimicking biological systems and exploiting quantum phenomena, respectively. These approaches offer the potential to process information in fundamentally different ways, enabling new computational capabilities.
Programming Models for Novel Architectures
The emergence of new computing paradigms necessitates the development of corresponding programming models. Current software development methodologies, built around the Von Neumann architecture, need adaptation to harness the unique characteristics of these emerging technologies. This includes developing new programming languages and frameworks tailored to these novel architectures. Examples of such languages and frameworks are currently under development and experimentation, tailored to the specifics of quantum computers or neuromorphic processors.
Data Representation and Management
Data representation plays a critical role in computational efficiency and accuracy. New computing paradigms may necessitate entirely new ways of representing and managing data, potentially leading to a shift from traditional numerical representations to more abstract or symbolic ones. This will necessitate a profound rethinking of data structures and algorithms. For example, the burgeoning field of graph databases is an illustration of this trend, emphasizing relationships between data rather than isolated values.
Comparison of Computing Models
Feature | Von Neumann Architecture | Neuromorphic Computing | Quantum Computing |
---|---|---|---|
Data Flow | Sequential, linear | Parallel, distributed | Probabilistic, superposition |
Processing Unit | Central Processing Unit (CPU) | Spiking Neural Networks | Qubits |
Memory | Separate from CPU | Integrated with processing elements | Potentially entangled qubits |
Programming Model | Imperative, procedural | Declarative, event-driven | Quantum gates, circuits |
This table highlights the key differences between the established Von Neumann model and emerging alternatives. The fundamental shifts in data flow, processing units, and programming models are indicative of a significant paradigm shift.
Implications for Software Development
The transition to new computing paradigms will profoundly impact software development. Software engineers will need to acquire new skills and knowledge to design, develop, and maintain applications that leverage these novel technologies. This will involve learning new programming languages, developing new algorithms, and adapting to the nuances of the new architectures. A crucial aspect will be the creation of new tools and frameworks to support these new paradigms.
Emerging Applications and Impacts
The post-Moore’s Law era, characterized by a shift beyond silicon, presents a landscape of unprecedented opportunities. Emerging technologies, such as neuromorphic computing and quantum computing, promise to revolutionize various sectors by enabling faster processing speeds, enhanced data analysis capabilities, and novel approaches to problem-solving. These advancements are not merely theoretical; real-world applications are beginning to emerge, promising transformative impacts across the globe.
Potential Applications Across Sectors
The diverse capabilities of these emerging technologies will unlock applications across numerous sectors. Their impact will be significant, ranging from advancements in medical diagnoses to sophisticated financial modeling and even revolutionary transportation systems. The potential for these technologies to improve human lives and drive economic growth is immense.
Applications in Medicine
Emerging technologies are poised to revolutionize medical diagnostics and treatment. Neuromorphic computing, with its ability to mimic the human brain’s neural networks, can accelerate drug discovery and personalize treatment plans. Quantum computing can simulate complex biological processes, leading to breakthroughs in understanding diseases like cancer and Alzheimer’s.
Applications in Finance
Quantum algorithms can analyze vast financial datasets to identify patterns and predict market trends with unprecedented accuracy. This allows for more sophisticated risk management and investment strategies, potentially leading to significant improvements in portfolio management. Neuromorphic systems can improve fraud detection and credit risk assessment by identifying subtle patterns in transactions that traditional methods might miss.
Applications in Transportation
Autonomous vehicles, powered by advanced computing capabilities, are rapidly evolving. Neuromorphic computing could enhance the real-time decision-making capabilities of self-driving cars, enabling them to react more effectively to complex and dynamic road conditions. Quantum computing can optimize traffic flow, potentially reducing congestion and improving overall transportation efficiency.
Innovative Uses Currently Being Explored
Numerous innovative applications are being explored across various sectors. For instance, researchers are investigating the use of neuromorphic chips for real-time image analysis in medical imaging, enabling faster and more accurate diagnoses. In finance, quantum algorithms are being tested for portfolio optimization, demonstrating the potential for increased returns and reduced risk. Companies are exploring quantum simulations for drug discovery and material science, potentially leading to the development of novel drugs and advanced materials.
Economic and Societal Impacts
These advancements will undoubtedly have significant economic and societal impacts. Increased productivity, improved decision-making, and new business models will reshape industries and create new job opportunities. However, the integration of these technologies also necessitates careful consideration of ethical implications, workforce retraining, and potential societal disruptions.
Projected Timelines for Adoption
The timelines for adoption and integration vary based on the specific technology and its application. Neuromorphic computing is expected to see significant progress in the next 5-10 years, with initial applications in niche areas and subsequent broader adoption following. Quantum computing, due to its inherent complexities, might require a longer timeframe, potentially 10-20 years, before reaching widespread use in mainstream applications.
Ethical Considerations and Challenges
Ethical considerations are crucial in the development and deployment of these technologies. Concerns regarding data privacy, algorithmic bias, and potential job displacement need careful attention. Robust regulations and ethical guidelines are essential to ensure responsible innovation and mitigate potential risks.
Potential Applications Table
Sector | Application | Benefits |
---|---|---|
Medicine | Personalized treatment plans, drug discovery, medical imaging analysis | Improved patient outcomes, faster diagnosis, reduced costs |
Finance | Risk assessment, portfolio optimization, fraud detection | Increased returns, reduced risk, improved decision-making |
Transportation | Autonomous vehicles, optimized traffic flow | Improved safety, reduced congestion, enhanced efficiency |
Challenges and Opportunities
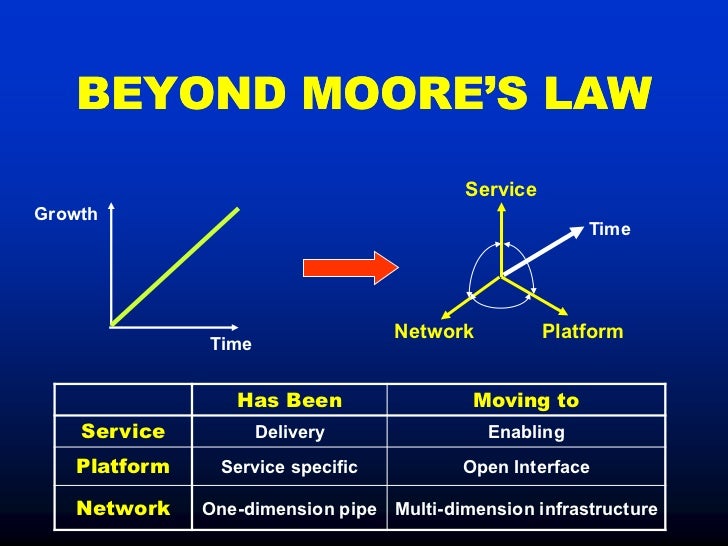
The transition beyond silicon presents a unique confluence of challenges and opportunities. While the limitations of silicon-based computing are becoming increasingly apparent, the path to alternative technologies is fraught with hurdles. Successfully navigating this transition requires a deep understanding of the obstacles, a clear vision of the opportunities, and a robust collaborative approach. The journey beyond silicon will not be linear, and requires careful consideration of the interplay between technological advancements, infrastructure development, and societal implications.The pursuit of post-silicon computing necessitates a paradigm shift in our understanding of hardware, software, and the entire computing ecosystem.
This shift will require significant investments, innovative approaches, and a proactive approach to overcome the inherent difficulties in replacing a technology that has served humanity so well for decades.
Key Challenges in the Transition
The transition from silicon to alternative technologies faces numerous hurdles. These challenges range from the development of novel materials and architectures to the creation of new fabrication processes and the development of accompanying software and algorithms. Addressing these obstacles requires a concerted effort from researchers, engineers, and policymakers.
- Material limitations and scalability: Developing new materials with the necessary properties, such as high conductivity, low power consumption, and high speed, is a significant challenge. Successfully scaling the production of these materials to meet the demands of mass manufacturing is equally important. Examples include the difficulty in large-scale production of graphene or the unique requirements of quantum computing materials, which can only be synthesized under specific conditions.
- Complex fabrication processes: Creating new architectures and components requires innovative fabrication techniques. The complexity of these processes often exceeds the capabilities of current manufacturing infrastructure. Existing semiconductor manufacturing processes are highly sophisticated, and developing comparable or better methods for new materials is crucial.
- Software and algorithm development: New hardware necessitates new software and algorithms. Existing software frameworks and tools are not always adaptable to these new technologies, requiring significant research and development efforts. Developing software and algorithms tailored to specific alternative architectures will be a key part of this transition.
- High development costs and long timelines: Research and development in post-silicon technologies is inherently expensive and time-consuming. Developing novel materials, designing new architectures, and validating these designs often takes years of effort and considerable financial investment.
Infrastructure, Expertise, and Funding
Widespread adoption of post-silicon technologies hinges on the availability of adequate infrastructure, expertise, and funding. Building the necessary infrastructure requires significant investment in research facilities, manufacturing plants, and training programs.
- Investment in research facilities: Supporting cutting-edge research requires substantial investments in state-of-the-art facilities equipped with the tools and instruments necessary for material characterization, device fabrication, and performance testing. National labs and university research centers will be essential.
- Developing a skilled workforce: The development and deployment of post-silicon technologies require a skilled workforce possessing expertise in new materials, architectures, fabrication techniques, and algorithms. Educational institutions need to adapt curricula to meet these evolving needs.
- Attracting funding and investment: Securing adequate funding from both public and private sources is critical for supporting research, development, and infrastructure. Attracting investment in emerging technologies requires showcasing clear potential returns and highlighting the long-term benefits of the technology.
Opportunities for Innovation and Progress
The transition beyond silicon presents unprecedented opportunities for innovation and progress. These opportunities span a wide range of applications, from enhanced computing power and efficiency to novel functionalities.
- Enhanced computing power: Alternative technologies offer the potential for significantly improved computing power and performance, enabling advancements in various fields such as artificial intelligence, scientific modeling, and data analysis. Quantum computing, for example, holds the promise of solving problems intractable for classical computers.
- New functionalities: Post-silicon technologies may unlock new functionalities and capabilities not possible with silicon. This includes advanced sensing, improved energy efficiency, and entirely new applications. Neuromorphic computing, with its biological inspiration, aims to create systems that mimic the human brain.
- Improved energy efficiency: Many post-silicon technologies have the potential to consume significantly less energy compared to silicon-based counterparts. This is particularly important for sustainable computing and resource management.
Collaborative Efforts
Collaboration between academia, industry, and government is crucial for successful transition. This collaboration can foster knowledge sharing, resource pooling, and the development of a robust ecosystem.
- Joint research projects: Collaborative research projects can combine the specialized knowledge and resources of different institutions. Joint projects between academia and industry can accelerate the development of new technologies.
- Government funding and support: Government funding can help accelerate research and development, provide crucial infrastructure support, and foster a supportive regulatory environment.
- Industry partnerships: Partnerships between industry leaders and research institutions can facilitate the translation of research findings into practical applications and commercial products. These partnerships can leverage the expertise of different players and accelerate the deployment of innovative technologies.
Transition Challenges and Opportunities
Obstacles | Opportunities |
---|---|
Material limitations and scalability | Enhanced computing power and new functionalities |
Complex fabrication processes | Improved energy efficiency and novel applications |
Software and algorithm development | Economic growth and job creation |
High development costs and long timelines | Advancements in scientific modeling and data analysis |
Future of Computing
The relentless march of technological advancement, beyond the limitations of Moore’s Law, promises a future of computing unlike anything we’ve experienced. The shift from silicon-based processors to novel architectures, coupled with the increasing sophistication of algorithms and software, will redefine the boundaries of what’s possible in computation. This evolution will not only impact individual lives but also reshape entire industries and societies.The future of computing hinges on our ability to overcome the physical constraints of silicon and explore new materials and paradigms.
This necessitates a fundamental shift in our approach to hardware design and software development, demanding innovation across multiple disciplines. This transformation will have significant implications for various sectors, from healthcare to finance, and will undoubtedly change how we interact with technology.
Potential Breakthroughs in the Next Decade
Emerging technologies, including quantum computing, neuromorphic computing, and advanced materials, hold immense promise for accelerating computation. Quantum computers, leveraging the principles of quantum mechanics, are poised to solve problems intractable for classical computers, opening up avenues for drug discovery, materials science, and financial modeling. Neuromorphic computing, inspired by the human brain, promises to achieve unprecedented efficiency in handling complex data patterns, impacting areas like artificial intelligence and machine learning.
Evolution of Computing Hardware
The transition from silicon-based processors to alternative architectures will be gradual, with various technologies coexisting and complementing each other. The timeline and specific adoption rates remain uncertain, but the trajectory suggests a multifaceted approach.
- Quantum Computing: Early-stage quantum computers are already tackling specific niche problems, demonstrating their potential. These computers, relying on quantum phenomena like superposition and entanglement, can theoretically outperform classical computers in certain tasks. However, scaling and error correction remain significant challenges. Examples include the development of quantum algorithms for drug discovery by companies like IBM and Google.
- Neuromorphic Computing: Inspired by the human brain, neuromorphic chips are designed to mimic the neural network architecture. This approach promises to achieve remarkable energy efficiency in tasks like pattern recognition and machine learning. Companies like Intel and IBM are exploring this area, focusing on applications like image recognition and speech processing.
- Advanced Materials: Novel materials, including graphene, carbon nanotubes, and other two-dimensional materials, offer the potential for faster and more energy-efficient transistors. These materials, with unique electronic properties, can lead to breakthroughs in high-speed computing and low-power consumption. Examples include research into graphene transistors and their potential applications in high-speed data transfer.
Impacts on Industries and Society
The evolution of computing will transform numerous industries, creating new job opportunities and posing new societal challenges. The impact will be profound, affecting how we live, work, and interact with each other.
- Healthcare: Advancements in computing will revolutionize medical diagnostics, personalized medicine, and drug discovery. More accurate and faster diagnostics, enabled by powerful computation, will lead to better treatment outcomes. AI-powered systems will analyze medical images and patient data to assist doctors in making faster and more informed decisions.
- Finance: High-frequency trading and complex financial modeling will benefit from the increased computational power. New algorithms and models will enable better risk management and portfolio optimization. Furthermore, the emergence of decentralized finance (DeFi) platforms will be facilitated by the ability to process transactions and manage assets quickly and efficiently.
- Manufacturing: The integration of advanced computing systems in manufacturing processes will lead to more efficient production lines, automated quality control, and optimized resource allocation. Smart factories, using real-time data analysis and predictive maintenance, will become increasingly common, improving productivity and reducing waste.
Long-Term Implications
The transition beyond Moore’s Law represents a paradigm shift in computing, influencing our understanding of information processing and its societal implications. This transition will continue to evolve, pushing the boundaries of computation and impacting various sectors.
Evolution of Computing Software
Software development will need to adapt to the new hardware architectures. Programming languages and methodologies will likely evolve to better leverage the unique capabilities of these technologies.
- Programming Languages: New languages tailored for quantum computing, neuromorphic computing, and other specialized architectures will emerge. Existing languages will adapt to incorporate new computational paradigms. The emergence of domain-specific languages (DSLs) will become more common for specialized applications.
- Software Development Methodologies: Agile methodologies and DevOps practices will become even more critical for managing the complexity of these emerging technologies. The ability to rapidly prototype, test, and deploy software will be essential to capitalize on the new capabilities.
Last Point: Life After Moores Law Beyond Silicon
In conclusion, the transition beyond silicon promises a future of unprecedented computing power and possibilities. While challenges remain, the potential benefits are enormous. From breakthroughs in medicine and finance to revolutionary advancements in transportation, the impact of this paradigm shift will be profound. This exploration has revealed the exciting possibilities of a future where computing is no longer limited by the constraints of silicon, but rather empowered by innovative alternatives.
The journey beyond Moore’s Law is just beginning, and its impact on our world is poised to be profound.