Plastic Discovery Means Advanced Memory
Plastic discovery means advanced memory sets the stage for a fascinating look into the future of memory technology. This innovative approach promises to revolutionize data storage, offering unprecedented capacity and speed, while potentially lowering costs compared to existing methods. We’ll explore the unique properties of plastic materials, delve into the intricate mechanisms of data storage, and examine the manufacturing processes involved.
The potential applications across various sectors, from electronics to healthcare, are truly remarkable. This exciting new field of research could reshape how we interact with information, driving progress in numerous industries.
The exploration will encompass a historical overview of memory technologies, highlighting their limitations and the promise of plastic-based alternatives. A comparative analysis, including tables showcasing key properties and performance metrics, will provide a comprehensive understanding of the potential of this innovative approach. We’ll also examine the challenges and future research directions in this burgeoning field, discussing potential solutions to overcome hurdles and pave the way for widespread adoption.
Introduction to Plastic Memory
Plastic memory, a burgeoning field in the realm of data storage, leverages the unique properties of polymers to create memory devices with potentially revolutionary capabilities. This new approach promises significant advancements in memory density, speed, and cost-effectiveness compared to existing technologies. This innovative approach draws inspiration from the adaptability of living organisms, where memory is encoded and stored in a dynamic and flexible manner.
The potential impact of plastic memory spans across diverse sectors, from mobile computing to artificial intelligence, revolutionizing how we store and process information.Existing memory technologies, such as DRAM and NAND flash, have dominated the market for decades. However, these technologies face limitations in terms of energy consumption, speed, and scalability. The quest for more efficient and powerful memory solutions has driven research into alternative materials and architectures, leading to the exploration of plastic memory.
Historical Context of Memory Research
Memory research has a long and rich history, marked by significant advancements. From the invention of the first electronic digital computer to the development of integrated circuits, each milestone has brought us closer to more efficient and compact memory storage. The quest for ever-increasing memory capacity, faster access times, and lower manufacturing costs has fueled ongoing research and innovation.
Key advancements include the invention of DRAM, the development of flash memory, and the exploration of novel materials like phase-change memory. These breakthroughs have collectively paved the way for the modern digital world.
Existing Memory Technologies and Their Limitations
Current memory technologies, while essential for modern computing, present limitations. DRAM, despite its high speed, suffers from high energy consumption and volatility, requiring constant power to retain data. NAND flash, though widely used in storage devices, exhibits limitations in terms of write endurance and potential for data degradation over time. These limitations drive the need for alternative solutions, such as plastic memory, that could address these shortcomings.
Potential Impact of Plastic Memory
The potential impact of plastic memory on various sectors is substantial. In mobile computing, plastic memory could enable ultra-thin and lightweight devices with extended battery life. In artificial intelligence, the high density and low cost of plastic memory could contribute to the development of more powerful and accessible AI systems. The potential for pervasive use in wearable technology and IoT devices is also substantial.
Comparison of Memory Technologies
Technology Type | Memory Capacity | Speed | Cost |
---|---|---|---|
DRAM | Moderate | High | Moderate |
NAND Flash | High | Moderate | Low |
Plastic Memory (Projected) | High (potentially exceeding NAND) | High (potentially exceeding DRAM) | Low (potentially lower than NAND) |
This table presents a comparison of key characteristics across different memory technologies. It is crucial to understand that projected values for plastic memory are still under development and subject to ongoing research and refinement.
Properties of Plastic Materials for Memory
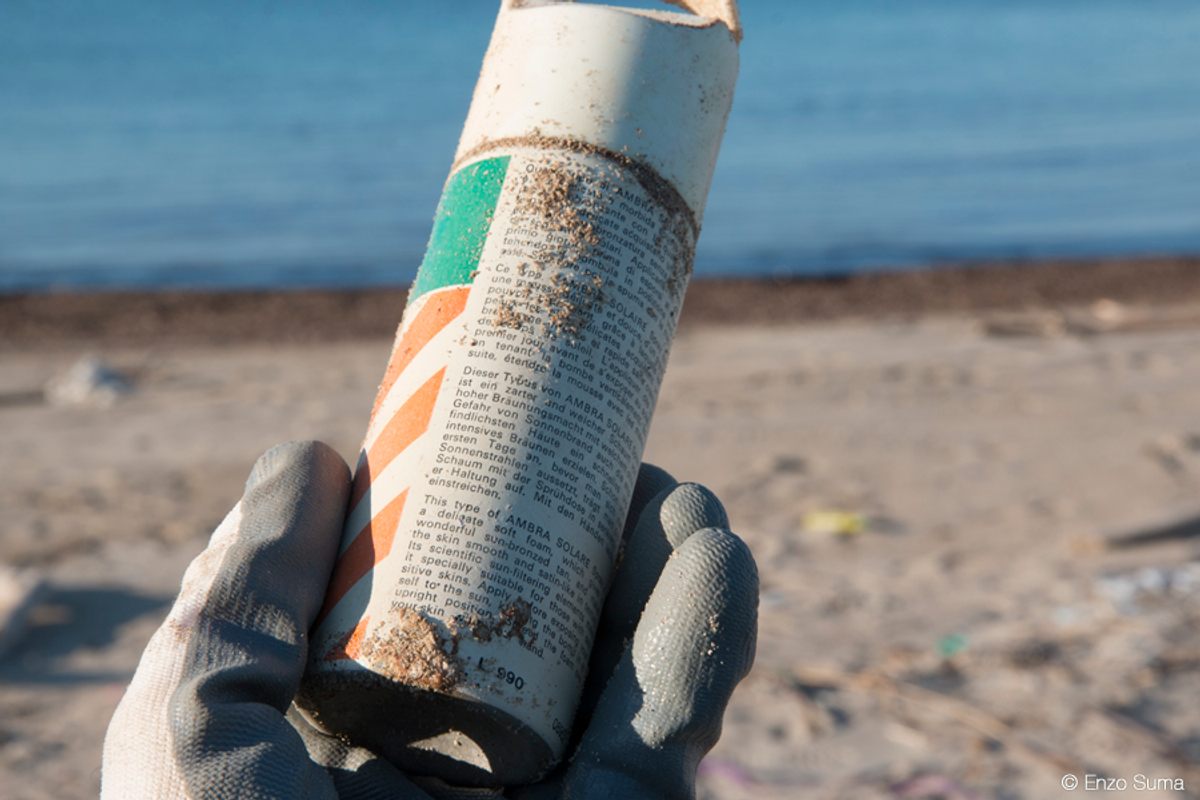
Plastic materials offer a unique blend of properties that make them attractive candidates for memory applications. Their ability to be molded into intricate shapes, combined with their relatively low cost and ease of processing, presents significant advantages over traditional memory technologies. This makes them a compelling area of research and development in the field of advanced memory systems.The suitability of plastic materials for memory applications hinges on their specific molecular structures.
These structures dictate how the material interacts with electrical signals, influencing the storage and retrieval of information. Understanding these relationships is crucial to optimizing plastic-based memory devices.
Recent discoveries in plastic science are hinting at the possibility of advanced memory storage. This could revolutionize data storage, potentially mirroring the advancements Microsoft is making in their next big move, Longhorn , which is promising a new era of data management. Ultimately, these innovations in plastic materials and data management will continue to push the boundaries of what’s possible in advanced memory technology.
Molecular Structure and Memory Function
The molecular structure of a plastic directly impacts its electrical conductivity and dielectric properties. These properties, in turn, are fundamental to how information is stored and retrieved in a memory device. For example, the presence of polar groups in the polymer chain can affect the material’s ability to store electrical charge, influencing its dielectric properties.
Advantages and Disadvantages of Different Plastic Types
Various types of plastics exhibit different properties, leading to varying advantages and disadvantages for memory applications. Polymers like polyvinyl chloride (PVC) and polyethylene terephthalate (PET) are known for their high dielectric strength and relatively low cost. However, their conductivity can be limited, potentially affecting the performance of the memory device. Conversely, polymers with conjugated double bonds, like poly(3,4-ethylenedioxythiophene) (PEDOT), show high conductivity but might have lower dielectric strength.
Performance Comparison of Plastic Types
The performance of different plastics in memory applications significantly depends on their electrical conductivity and dielectric properties. A higher conductivity allows for faster data transfer, while a higher dielectric constant facilitates greater charge storage. However, these properties are often intertwined, and optimizing both for a specific application is a key challenge.
Plastic Types and Their Properties in Memory Devices
Plastic type | Conductivity (S/m) | Dielectric constant | Temperature range (°C) |
---|---|---|---|
Polyethylene (PE) | Very low | 2.3 | -70 to +120 |
Polyvinyl chloride (PVC) | Low | 3.5 | -50 to +80 |
Polycarbonate (PC) | Very low | 3.0 | -100 to +150 |
Poly(3,4-ethylenedioxythiophene) (PEDOT) | High | 3.0-6.0 | -40 to +120 |
The table above provides a concise overview of the electrical conductivity, dielectric constant, and operating temperature ranges for various common plastic types. Note that these values can vary depending on the specific formulation and processing conditions of the plastic material. The conductivity values are given as an indication of the material’s ability to conduct electricity; a lower value indicates poorer conductivity.
Mechanisms of Data Storage in Plastic
Plastic materials, once primarily considered disposable, are now emerging as promising candidates for data storage. Their inherent properties, coupled with advancements in nanotechnology, offer unique possibilities for creating robust and potentially low-cost memory devices. This exploration delves into the mechanisms behind data storage in plastic, focusing on the manipulation of plastic structures for encoding information.
Recent discoveries in plastic science are promising, hinting at advanced memory capabilities. This exciting development could lead to incredible breakthroughs in tech, similar to how Sun’s Solaris update now offers an open source option, suns solaris update includes open source option , potentially paving the way for more accessible and innovative solutions. Ultimately, these advancements in plastic and software will drive further innovation in memory technology.
Different Mechanisms of Data Storage
Various methods exist for encoding information within plastic matrices. These techniques exploit the inherent properties of polymers, including their structural flexibility and the ability to undergo changes at the molecular level. Different types of plastic exhibit unique characteristics that lend themselves to specific storage mechanisms. For example, some plastics are more susceptible to changes in their optical properties when exposed to light, while others can be modified by incorporating specific nanoparticles.
Manipulating Plastic Structures for Data Encoding
Data encoding in plastic materials relies on altering the structure of the plastic itself. This can involve several techniques, each with its own advantages and limitations. One approach involves embedding nanoparticles or nanostructures within the plastic matrix. These inclusions can be engineered to alter the optical, electrical, or magnetic properties of the plastic. Another method involves creating localized changes in the polymer chain structure, such as crosslinking or grafting, which can impact the material’s mechanical or optical properties.
Data Reading and Writing Processes
The processes of reading and writing data in plastic memory devices vary based on the specific encoding method employed. Reading data often involves detecting changes in the material’s properties, such as changes in its refractive index, conductivity, or magnetic field. The read process may involve scanning the material with a laser beam, applying an electric field, or using a magnetic field sensor.
Conversely, the write process involves inducing the desired change in the plastic’s structure. This could involve exposure to specific wavelengths of light, applying an electrical current, or manipulating the material’s temperature.
Examples of Data Storage Methods in Plastic
Various examples illustrate the potential of plastic memory. One example involves incorporating light-sensitive molecules into a polymer matrix. Changes in light exposure alter the molecular structure, encoding information. Another example leverages the piezoelectric properties of certain polymers. Applying pressure to the plastic material creates a corresponding electrical signal, which can be used for data storage.
Furthermore, the incorporation of magnetic nanoparticles can enable magnetic-based storage.
Table of Data Storage Methods in Plastic
Storage method | Data encoding | Read process | Write process |
---|---|---|---|
Optical encoding | Changes in refractive index, light absorption | Laser scanning, detecting light scattering/transmission changes | Exposure to specific wavelengths of light |
Electrical encoding | Changes in conductivity, charge density | Applying an electric field, measuring current flow | Applying electric current, injecting charges |
Magnetic encoding | Magnetic alignment of nanoparticles | Detecting magnetic field changes | Applying magnetic field, manipulating nanoparticle alignment |
Mechanical encoding | Changes in physical structure, deformation | Measuring deformation, strain | Applying pressure, stretching |
Manufacturing Processes and Techniques: Plastic Discovery Means Advanced Memory
Plastic memory devices, with their potential for low cost and high scalability, are poised to revolutionize data storage. Understanding the manufacturing processes is crucial to realizing this potential. This involves careful selection of plastic materials, precise control over fabrication steps, and effective quality control measures. The scalability of these processes will determine the viability of widespread adoption.The fabrication of plastic memory chips involves a series of steps, starting from material preparation and progressing through various processing techniques.
Recent discoveries about plastics and their potential for advanced memory storage are fascinating. Think about how this could revolutionize data storage, and imagine the implications for everything from personal computers to massive data centers. Meanwhile, the VeriSign Wildcard Service has sparked a call to halt a lawsuit, a significant development in the domain name industry. This VeriSign Wildcard Service action could potentially impact how we think about online security and domain name management.
Ultimately, the future of memory storage, possibly with plastic-based advancements, seems very promising.
The precision and consistency of these steps are paramount to achieving reliable data storage and retention. Challenges associated with scaling up plastic memory production, such as maintaining uniform material properties and achieving high yields, are being actively addressed by researchers and engineers. Addressing these issues is vital for the commercial viability of this technology.
Plastic Material Selection
Plastic materials with specific properties are chosen for their compatibility with the desired memory functionality and manufacturing processes. This selection process involves evaluating factors such as dielectric properties, mechanical strength, and thermal stability. Careful consideration is given to minimizing material defects and maximizing the desired electrical properties for data storage. This selection process directly impacts the performance and reliability of the resulting plastic memory devices.
Fabrication Steps in Plastic Memory Chips, Plastic discovery means advanced memory
- Material Preparation: Raw plastic materials undergo purification and preparation to ensure uniformity in their composition and properties. This involves precise measurements of additives and careful mixing to maintain consistent quality across batches.
- Molding: Molded plastic structures serve as the foundation for the memory components. Precise molding techniques, like injection molding or 3D printing, are essential to create complex patterns and shapes for the data storage elements. Advanced molding techniques are constantly evolving to improve accuracy and speed.
- Pattern Transfer: Transferring intricate patterns onto the plastic substrate is crucial for data storage. This step can involve lithography techniques, where a pattern is created using light or other forms of energy. Different techniques, such as nanoimprint lithography or laser ablation, are explored to define the memory cells with the desired precision and resolution.
- Functionalization: This step involves applying functional materials to the plastic structures to enhance their electrical properties and improve data retention. For example, doping with specific elements or the deposition of conductive layers are crucial for efficient data storage and retrieval. The application of these materials directly impacts the performance characteristics of the memory device.
- Assembly: The final stage involves assembling the individual components into a functional plastic memory chip. Automated assembly techniques are crucial for achieving high throughput and maintaining consistent quality across the production process. This process is vital to ensuring that the final device functions as intended.
Scaling Up Plastic Memory Production
Scaling up plastic memory production presents significant challenges. Maintaining consistent material properties across large production runs is crucial. Implementing automated manufacturing processes and reducing production costs are key objectives. High-volume manufacturing techniques, such as those employed in semiconductor production, are being investigated to streamline the process. Improving yield rates and minimizing defects is paramount to cost-effective production.
Methods for Improving Manufacturing Efficiency
- Automation: Implementing automated processes for material handling, molding, and assembly can significantly improve efficiency and reduce production costs. Automation reduces human error and allows for higher throughput.
- Optimization of Molding Techniques: Optimizing molding techniques to reduce cycle times and improve the quality of molded parts is essential. This includes exploring new materials and refining existing molding methods to reduce waste and improve consistency.
- Advanced Lithography: Using advanced lithography techniques for pattern transfer can increase precision and reduce the size of memory cells, potentially leading to higher density and faster speeds.
- Improved Quality Control: Implementing robust quality control measures at each stage of production can minimize defects and improve yield rates. This includes using advanced metrology tools to monitor the quality of materials and components.
Fabrication Process Table
Fabrication Stage | Materials Used | Equipment | Quality Control |
---|---|---|---|
Material Preparation | Plastic resins, additives | Mixers, purifiers | Composition analysis, particle size distribution |
Molding | Plastic resins, molds | Injection molding machines, 3D printers | Dimensional accuracy, surface finish |
Pattern Transfer | Photoresists, masks | Lithography equipment, laser systems | Pattern fidelity, defect density |
Functionalization | Conductive materials, dopants | Deposition systems, coating tools | Film thickness, electrical conductivity |
Assembly | Interconnects, packaging materials | Automated assembly systems | Electrical continuity, mechanical integrity |
Applications and Potential Uses
Plastic memory, with its unique properties and potential, promises a paradigm shift in various sectors. Its inherent advantages, including low cost, flexibility, and potential for large-scale manufacturing, could lead to a significant decrease in the price of memory storage and an increase in its accessibility. The ability to tailor plastic memory to different needs opens doors to novel applications, exceeding the limitations of existing technologies.
Potential Applications in Electronics
Plastic memory’s flexibility and low cost make it a compelling alternative for diverse electronic devices. Imagine flexible displays, wearable electronics, and even foldable smartphones, all benefiting from the inherent adaptability of plastic memory. This opens doors for previously inconceivable designs, potentially revolutionizing the form factor and functionality of everyday electronics. Moreover, the integration of plastic memory in microelectronics could result in smaller, lighter, and more energy-efficient devices.
Applications in Healthcare
The potential applications of plastic memory in healthcare are equally profound. Biosensors embedded within implantable devices, offering continuous health monitoring, could be a game-changer in patient care. The ability to create miniature, biocompatible plastic memory devices paves the way for long-term, non-invasive monitoring of vital signs, enabling proactive interventions and improving overall health outcomes.
Applications in Data Storage
In the realm of data storage, plastic memory’s potential to provide high-density storage at a lower cost is substantial. Imagine archiving vast amounts of data on flexible plastic sheets, a paradigm shift from current rigid storage media. This could dramatically reduce the cost of data storage, making it more accessible for individuals and organizations. Furthermore, the durability and longevity of plastic memory, when combined with appropriate protective coatings, could offer a secure and reliable solution for long-term data retention.
Revolutionizing Existing Technologies
Plastic memory has the potential to revolutionize existing technologies in several key areas. In electronic devices, it could enable more flexible and adaptable designs, leading to smaller, lighter, and more portable devices. In healthcare, continuous health monitoring and long-term data storage become more feasible. In data storage, it could provide a more cost-effective and scalable alternative to traditional methods.
Addressing Limitations of Existing Memory Technologies
Existing memory technologies often face limitations in terms of scalability, cost, and flexibility. Plastic memory, with its potential for large-scale manufacturing and inherent flexibility, could directly address these challenges. The ability to integrate plastic memory into diverse applications and tailor its properties opens new avenues for innovation.
Application | Benefit | Current limitations | Plastic memory solution |
---|---|---|---|
Flexible Displays | Lightweight, bendable displays | Current displays are rigid and can be brittle. | Plastic memory enables flexible displays, opening up new design possibilities. |
Wearable Electronics | Lightweight and comfortable devices | Current wearable electronics are often bulky and power-hungry. | Plastic memory enables the creation of smaller, lighter, and more energy-efficient wearable devices. |
Implantable Biosensors | Continuous health monitoring | Current biosensors often require frequent replacements. | Plastic memory allows for the creation of miniature, biocompatible, long-term biosensors. |
High-Density Data Storage | Cost-effective and scalable data storage | Current storage media often face limitations in density and cost. | Plastic memory offers a scalable and cost-effective solution for high-density data storage. |
Challenges and Future Directions
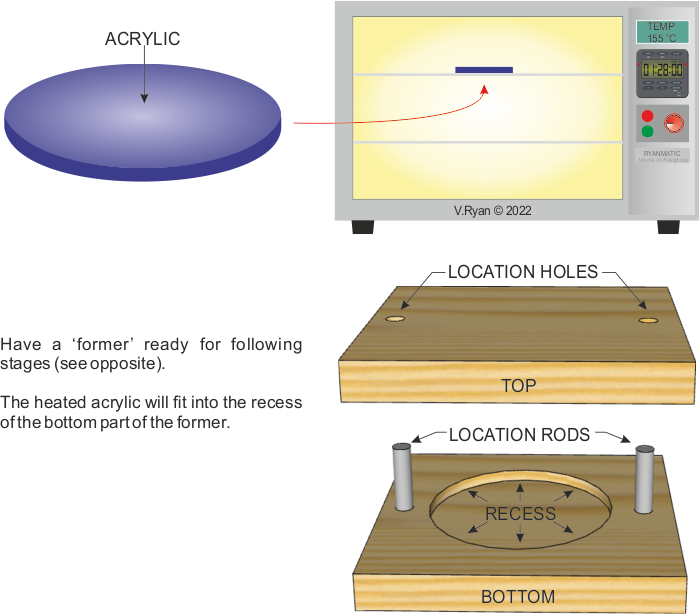
Plastic memory, while promising, faces hurdles that need careful consideration. These limitations, if overcome, could lead to a paradigm shift in data storage, but require substantial research and development. The journey from laboratory prototypes to widespread commercial applications is paved with challenges that must be addressed.
Material Limitations and Degradation
Plastic materials, despite their versatility, are not inherently stable. Their susceptibility to degradation from environmental factors like heat, moisture, and light can severely impact data retention and reliability. Furthermore, achieving consistent material properties across large-scale manufacturing remains a significant hurdle.
- Material Degradation: The chemical and physical breakdown of the plastic matrix can alter the properties that support data storage, leading to data loss or corruption over time. This degradation can be accelerated by exposure to extreme temperatures, UV radiation, or specific chemicals.
- Material Variability: Variations in the raw materials, manufacturing processes, and environmental conditions can lead to inconsistencies in the performance and reliability of plastic memory devices. This poses a challenge for achieving standardized production and predictable results.
- Data Retention Longevity: The duration for which stored data remains intact is crucial. A shorter lifespan necessitates frequent rewrites or backups, diminishing the advantages of plastic memory compared to more durable alternatives.
Data Storage Density and Read/Write Speed
Increasing the density of data stored on a given area of plastic and improving the speed at which data can be written and read are critical for practical applications. Current methods often struggle to match the performance of traditional semiconductor-based memory.
- Density Limitations: Current techniques for embedding data within the plastic matrix may not be able to achieve the high density levels needed for storing large volumes of information. Improving this is essential for applications requiring significant data storage capacity.
- Read/Write Speed: The rate at which data can be accessed and modified is another key performance metric. Slow read/write speeds can hinder the practical usability of plastic memory in real-world applications.
- Scalability Issues: Scaling up the production of plastic memory devices to meet demand and maintain consistent quality poses significant challenges in manufacturing.
Cost-Effectiveness and Manufacturing Processes
The manufacturing process and cost of producing plastic memory devices are essential factors in determining their viability. Optimizing the production techniques and reducing costs are crucial for market penetration.
- Manufacturing Costs: Current manufacturing processes for plastic memory can be expensive, hindering widespread adoption. Finding cheaper and more efficient methods is crucial for affordability.
- Scalability of Production: The ability to ramp up production to meet demand is essential for making plastic memory a practical alternative to current technologies. Current processes may not be readily scalable for high-volume production.
Table: Challenges and Potential Solutions
Challenge | Current Solutions | Future Research Directions | Expected Impact |
---|---|---|---|
Material Degradation | Stabilizing additives, improved polymer selection | Developing self-healing polymers, incorporating protective coatings | Enhanced data retention, longer lifespan of devices |
Data Storage Density | Advanced nanomaterials, novel embedding techniques | Developing new data encoding methods, optimizing material structure | Increased storage capacity, improved performance in data-intensive applications |
Read/Write Speed | Optimizing the interaction of data signals with the plastic | Exploring new materials with enhanced conductivity, designing new data encoding methods | Improved speed and efficiency, better responsiveness in real-time applications |
Cost-Effectiveness | Recycling of plastic waste, developing cheaper manufacturing techniques | Developing new, more cost-effective production processes, exploring 3D printing methods | Wider accessibility and market penetration of plastic memory, potential for lower cost devices |
Outcome Summary
In conclusion, the discovery of plastic-based memory holds immense promise for transforming the way we store and access information. This technology could revolutionize numerous industries, offering a compelling solution to current limitations in memory technologies. The potential for high capacity, speed, and reduced cost makes plastic memory a truly exciting prospect for the future. While challenges remain, the potential rewards are substantial, promising a new era of memory innovation.